Emerging Diseases Research Group, University of Georgia, College of Veterinary Medicine
Athens, GA, USA
Abstract
Superficial microbial infections remain a common cause of morbidity and mortality in aquatic species. Equally concerning is the development and subsequent maintenance of multi-drug resistant pathogens in the water column. Based on research to develop methods of controlling multi-drug resistant bacteria in human burn patients we have formulated an antimicrobial potentiator that facilitates the killing of bacteria and fungi (irrespective of their resistance profile), promotes wound healing and appears to prevent microbes from developing resistance to the antimicrobial agents with which it is mixed. Our in vitro and in vivo experimental data as well as subjective case material concerning the clinical use of this antimicrobial potentiator will be presented.
Introduction
The repair of damaged skin requires an ongoing process of physiologic debridement, development of highly vascular granulation tissue that provides wound protection, wound closure through contraction and epithelial migration to provide long-term surface protection. The interval between wounding and skin repair depends on the location of the injury, the degree of damage, the apposition of the wound edges, the medical condition of the patient and the type and severity of wound contamination with foreign debris and microbes.
Intact epithelium provides a critical barrier to fluid loss and prevents the underlying tissues from being invaded by a plethora of environmental bacteria and fungi, including the commensal organisms that inhabit the outer layers of the skin. Once a wound occurs, the damaged tissue along with any deposited foreign matter provides a warm, moist, nutrient rich environment for microbial proliferation.
Trauma and necrosis of the skin decreases vascularization to a wound and thus slows the influx of immunologic proteins and white blood cells. The severity of the damage and number of invading microbes determine whether or not a clinical infection occurs. While clinicians frequently focus on the type of microbes that may contaminate a wound, some studies suggest that the number of invading microbes is more important than the species [1]. Proliferating microbes cause additional and accelerated tissue damage through both direct (toxins and cellular damage) and indirect (edema and accumulation of pus) impairment of vascular supply. These changes further impair access of immune system components to the wound as well as reducing the clearance of necrotic debris and preventing systemically delivered antibiotics from reaching contaminated tissues. Collagenase and proteases that accumulate in association with degenerating inflammatory cells damage connective tissue proteins and further inhibit wound healing [2].
The wound healing cascade is delayed until the inflammatory and physiologic debridement phases have killed and removed contaminating microbes and necrotic tissues [3]. Experiments in rats demonstrated that infection decreased the bursting strength of a wound (increased likelihood of dehiscence) as well as increasing angiogenesis and vessel thrombosis [4]. While some data suggests infected wounds that heal by second intention are stronger than ones closed primarily, it is generally considered best to resolve and prevent infection [5].
Macrophages that enter a wound serve a phagocytic function as well as the critical role of stimulating the maturation of fibroblast that are ultimately responsible for skin repair. Epithelial cell proliferation and migration are necessary for re-epithelization. This process is enhanced in a moist environment that is well oxygenated by a viable blood supply.
Infection and Wound Healing
Initiating treatment in a timely manner is most important to reduce or prevent microbial colonization and additional pathogen-associated wound damage. Contaminating bacteria begin to invade adjacent tissue within 6 hours and most data suggest that a wound should be considered infected when 105 to 107 microbes are present per gram of tissue [6]. Solutions containing antimicrobials have been used for flushing wounds [7,8]. However, the persistent use of antimicrobials or antiseptics can result in the proliferation of multi-drug resistant bacteria.
Because of these concerns, traditional wound therapies may prove to be inferior to solutions containing the third generation chelating agent, Tricide (Molecular Therapeutics, Riverbend Laboratories, Athens, GA, 706-542-7235), either alone or when mixed with antibiotics or antiseptics. Solutions containing the antibiotic potentiator Tricide have been shown to be effective against multi-drug-resistant bacteria ("flesh eating bacteria") cultured from human burn patients and from companion animals [9]. This potentiator can be prepared with normal saline, is stable to sterilization (121 C for 20 minutes) or micropore filtration, has a long shelf life, mixes well with multiple antibiotics and antifungals, makes many otherwise resistant gram-positive and gram-negative bacteria, as well as fungi and yeast, susceptible to low class antimicrobials (Table 1), reduces the concentration of antimicrobials necessary to achieve an MIC and MBC, requires relatively short contact times (5 to 10 minutes) and has been shown to decrease the ability of a treated bacteria to develop resistance to either the potentiator or the antimicrobial with which it is mixed [9] This latter fact could be of untold benefit in reducing the development of multi-drug resistant bacteria in aquatic environments.
While as yet unavailable for use in humans, Tricide is available to the veterinary community for treating superficial bacterial infections in companion fish, particularly cutaneous ulcerations (ulcer disease) (Figure 1).
Click on the image to see a larger view
Figure 1. | 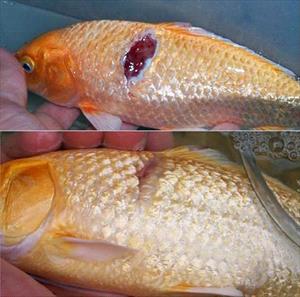
|
|
| |
Mechanisms of Antibiotic Potentiation
The antimicrobial action of antibiotics varies by class of medicant. As examples, penicillins damage the bacterial cell wall, polymyxin B effects the cytoplasmic membrane, oxytetracycline, chloramphenicol, streptomycin and gentamicin inhibit protein synthesis at the ribosomal level, nalidixic acid inhibits DNA formation and sulfonamides block intermediary metabolic pathways. The chelating agents found in Tricide have been shown to potentiate the antimicrobial effects of all these groups of antibiotics, irrespective or their mode of action [10, 11]. Tricide has a rather interesting mode of activity that facilitates its spectrum of activity. Tricide creates holes (Figure 2) in the bacterial cell wall which in-turn allows antibiotics to flood the organism, destroys the effectiveness of the bacteria's efflux pump and facilitates osmotic collapse of the bacterium. Because the potentiator causes direct physical damage to the bacteria, one is able to reduce the likelihood that exposed bacteria will develop resistance to the chosen antibiotic.
Click on the image to see a larger view
Figure 2. | 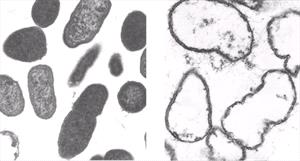 Pseudomonas sp. before and after exposure to Tricide-Neo. Normal "intact" cell wall before exposure Tricide induced cytoplasmic leakage . |
|
| |
Continued concerns about the emergence and environmental persistence of anitmicrobial resistant organisms suggests the importance of using therapies that reduce the evolution of these organisms. Gram-positive and gram-negative bacteria treated with Tricide potentiated antibiotic solutions were not shown to develop resistance to the tested antibiotics compared to control bacterium (Table 2). If Tricide mixed with low class antimicrobials were used in place of antibiotic preparations alone for treatment of all superficial and mucosal infections, one could theorize that the evolution of drug-resistant bacteria may be slowed reserving high class antibiotics for the treatment of systemic infections.
In companion fish, Tricide mixed with antibiotics has been shown to potentiate the wound healing process, in an as yet undefined manner, that must involve a mechanism of action other than its defined antimicrobial activity. The Tricide with neomycin treatment protocol for fish with ulcer disease involves a 5 minute bath every day to every other day after which the treated fish is placed in water that does not contain the treatment solution. One would assume that Tricide and neomycin present on the fish is diluted to a level below its MIC soon after the fish is removed from the treatment solution, yet healing of the ulcer proceeds.
Table 2. Effects on antibiotic resistance of serial passages of Staphylococcus aureus, Pseudomonas aeruginosa, Escherichia coli and Proteus mirabilis in Neomycin, Oxytetracycline, Tricide alone and Tricide mixed with Neomycin or Oxytetracycline.
Sensitive bacterium were serial passaged 5 times in test solution or until resistance developed. Intermediate sensitivity in the laboratory is considered clinically resistant. Note that the addition of Tricide made resistant forms of bacteria sensitive and no detectable resistance developed to Tricide, Tricide with Neomycin or Tricide with Oxytetracycline after 5 passages. Sensitive (Sen), Resistant (Res), Intermediate (Int), Neomycin (Neo), Oxytetracyline (Oxytet).
Organism |
% Decrease
Neomycin |
Staphylococcus aureus |
96 |
Streptococcus iniae |
99 |
Aeromonas hydrophila |
50 |
Pseudomonas aeruginosa |
99 |
Disclosure
The authors are employees of the University of Georgia and are inventors of antibiotic potentiator technology marketed under the name Tricide. The rights to the technology marketed under the name Tricide were licensed by the University of Georgia Research Foundation (UGARF) to Molecular Therapeutics, which pays a royalty to UGARF for rights to the technology.
References
1. Mader JT, Cierny G. The principles of the use of preventative antibiotics. Clin Orthop 1984; 190:75.
2. Swaim SF. Wound healing. In: Swaim SF, editor. Surgery of traumatized skin: Management and reconstruction in the dog and cat. Philadelphia; WB Saunders; 1980. p. 70.
3. Peacock EE, Van Winkle W. Wound repair. Philadelphia, WB Saunders, 1984, p 1-141.
4. Bucknall TE. The effect of local infection upon wound healing: An experimental study. Br J Surg 1980; 67:851.
5. Johnson BW, Scott PG, Brunton JL. Primary and secondary healing in infected wounds. Arch Surg 1982; 117:1189.
6. Edlich RF, Rodeheaver GT, Thacker JG. Technical factors in the prevention of wound infections. In: Simmons RL, Howard RJ, editors. Surgical infectious diseases. New York: Appleton-Centure-Crofts, 1982. p. 449-72.
7. Lee AH, Swaim SF, Yang ST, Wilken LO. Effects of gentamicin solution and cream on the healing of open wounds. Am J Vet Res 1984; 45:1487-92.
8. Peyton LC. Wound healing in the horse. Comp Contin Ed 1987; 9:191-202.
9. Wooley RE, Ritchie BW, Burnley VV, Kemp, DT. The potentiation effect of Tricide on selected antimicrobial agents against pathogenic bacteria, fungi, and yeast isolated from dogs. Submitted for publication. Veterinary Medicine 2004.
10. Wooley RE, Jones MS. Action of EDTA-tris and antimicrobial agent combinations on selected pathogenic bacteria. Vet Microbiol 1983; 8:271-80.
11. Wooley RE, Ritchie BW, Burnley VV. In vitro effect of a buffered chelating agent and neomycin or oxytetracycline on bacteria associated with diseases of fish. Dis Aquat Org 2004; 59:263-267.